Abstract
Extracellular deposition of amyloid-β as neuritic plaques and intracellular accumulation of hyperphosphorylated, aggregated tau as neurofibrillary tangles are two of the characteristic hallmarks of Alzheimer’s disease1,2. The regional progression of brain atrophy in Alzheimer’s disease highly correlates with tau accumulation but not amyloid deposition3,4,5, and the mechanisms of tau-mediated neurodegeneration remain elusive. Innate immune responses represent a common pathway for the initiation and progression of some neurodegenerative diseases. So far, little is known about the extent or role of the adaptive immune response and its interaction with the innate immune response in the presence of amyloid-β or tau pathology6. Here we systematically compared the immunological milieux in the brain of mice with amyloid deposition or tau aggregation and neurodegeneration. We found that mice with tauopathy but not those with amyloid deposition developed a unique innate and adaptive immune response and that depletion of microglia or T cells blocked tau-mediated neurodegeneration. Numbers of T cells, especially those of cytotoxic T cells, were markedly increased in areas with tau pathology in mice with tauopathy and in the Alzheimer’s disease brain. T cell numbers correlated with the extent of neuronal loss, and the cells dynamically transformed their cellular characteristics from activated to exhausted states along with unique TCR clonal expansion. Inhibition of interferon-γ and PDCD1 signalling both significantly ameliorated brain atrophy. Our results thus reveal a tauopathy- and neurodegeneration-related immune hub involving activated microglia and T cell responses, which could serve as therapeutic targets for preventing neurodegeneration in Alzheimer’s disease and primary tauopathies.
This is a preview of subscription content, access via your institution
Access options
Subscribe to this journal
Receive 51 print issues and online access
199,00 € per year
only 3,90 € per issue
Rent or buy this article
Get just this article for as long as you need it
$39.95
Prices may be subject to local taxes which are calculated during checkout
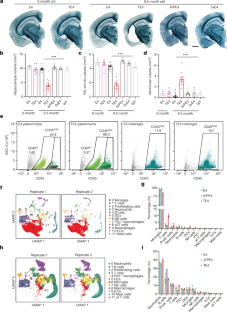
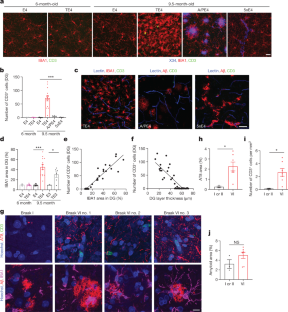
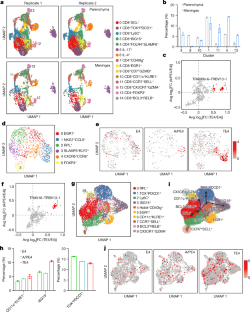
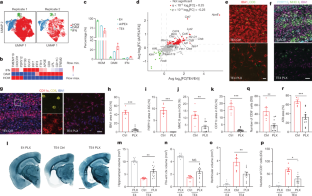
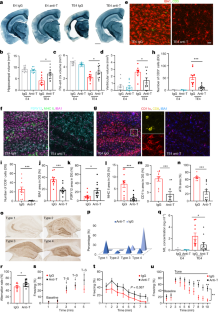
Data availability
Immune scRNA-seq sample information, information for samples from patients with Alzheimer’s disease, and immune cell numbers in each cluster in the brain are available in the Supplementary Information. All source data, including sequencing reads and single-cell expression matrices, are available from the Gene Expression Omnibus under accession code GSE221856.
Code availability
Code for preprocessing of immune scRNA-seq bioinformatic analysis is available at https://zenodo.org/record/7566414.
References
-
Duyckaerts, C., Delatour, B. & Potier, M. C. Classification and basic pathology of Alzheimer disease. Acta Neuropathol. 118, 5–36 (2009).
-
Masters, C. L. et al. Alzheimer’s disease. Nat. Rev. Dis. Primers 1, 15056 (2015).
-
Jack, C. R. Jr & Holtzman, D. M. Biomarker modeling of Alzheimer’s disease. Neuron 80, 1347–1358 (2013).
-
Musiek, E. S. & Holtzman, D. M. Three dimensions of the amyloid hypothesis: time, space and ‘wingmen’. Nat. Neurosci. 18, 800–806 (2015).
-
Giannakopoulos, P. et al. Tangle and neuron numbers, but not amyloid load, predict cognitive status in Alzheimer’s disease. Neurology 60, 1495–1500 (2003).
-
Chen, X. & Holtzman, D. M. Emerging roles of innate and adaptive immunity in Alzheimer’s disease. Immunity 55, 2236–2254 (2022).
-
Ulrich, J. D. et al. Altered microglial response to Aβ plaques in APPPS1-21 mice heterozygous for TREM2. Mol. Neurodegener. 9, 20 (2014).
-
Xiong, M. et al. APOE immunotherapy reduces cerebral amyloid angiopathy and amyloid plaques while improving cerebrovascular function. Sci. Transl. Med. 13, eabd7522 (2021).
-
Rupp, N. J., Wegenast-Braun, B. M., Radde, R., Calhoun, M. E. & Jucker, M. Early onset amyloid lesions lead to severe neuritic abnormalities and local, but not global neuron loss in APPPS1 transgenic mice. Neurobiol. Aging 32, 2324 (2011).
-
Giannoni, P. et al. Cerebrovascular pathology during the progression of experimental Alzheimer’s disease. Neurobiol. Dis. 88, 107–117 (2016).
-
Yoshiyama, Y. et al. Synapse loss and microglial activation precede tangles in a P301S tauopathy mouse model. Neuron 53, 337–351 (2007).
-
Long, J. M. & Holtzman, D. M. Alzheimer disease: an update on pathobiology and treatment strategies. Cell 179, 312–339 (2019).
-
Hammond, T. R., Marsh, S. E. & Stevens, B. Immune signaling in neurodegeneration. Immunity 50, 955–974 (2019).
-
Heneka, M. T., Kummer, M. P. & Latz, E. Innate immune activation in neurodegenerative disease. Nat. Rev. Immunol. 14, 463–477 (2014).
-
Krasemann, S. et al. The TREM2-APOE pathway drives the transcriptional phenotype of dysfunctional microglia in neurodegenerative diseases. Immunity 47, 566–581 (2017).
-
Mrdjen, D. et al. High-dimensional single-cell mapping of central nervous system immune cells reveals distinct myeloid subsets in health, aging, and disease. Immunity 48, 380–395 (2018).
-
Prinz, M., Priller, J., Sisodia, S. S. & Ransohoff, R. M. Heterogeneity of CNS myeloid cells and their roles in neurodegeneration. Nat. Neurosci. 14, 1227–1235 (2011).
-
Fischer, H. G. & Reichmann, G. Brain dendritic cells and macrophages/microglia in central nervous system inflammation. J. Immunol. 166, 2717–2726 (2001).
-
Gate, D. et al. CD4+ T cells contribute to neurodegeneration in Lewy body dementia. Science 374, 868–874 (2021).
-
Zenaro, E. et al. Neutrophils promote Alzheimer’s disease-like pathology and cognitive decline via LFA-1 integrin. Nat. Med. 21, 880–886 (2015).
-
Subbarayan, M. S., Hudson, C., Moss, L. D., Nash, K. R. & Bickford, P. C. T cell infiltration and upregulation of MHCII in microglia leads to accelerated neuronal loss in an alpha-synuclein rat model of Parkinson’s disease. J. Neuroinflammation 17, 242 (2020).
-
Garber, C. et al. T cells promote microglia-mediated synaptic elimination and cognitive dysfunction during recovery from neuropathogenic flaviviruses. Nat. Neurosci. 22, 1276–1288 (2019).
-
Gate, D. et al. Clonally expanded CD8 T cells patrol the cerebrospinal fluid in Alzheimer’s disease. Nature 577, 399–404 (2020).
-
Merlini, M., Kirabali, T., Kulic, L., Nitsch, R. M. & Ferretti, M. T. Extravascular CD3+ T cells in brains of Alzheimer disease patients correlate with Tau but not with amyloid pathology: an immunohistochemical study. Neurodegener. Dis. 18, 49–56 (2018).
-
Laurent, C. et al. Hippocampal T cell infiltration promotes neuroinflammation and cognitive decline in a mouse model of tauopathy. Brain 140, 184–200 (2017).
-
Lee, S. H. et al. TREM2-independent oligodendrocyte, astrocyte, and T cell responses to tau and amyloid pathology in mouse models of Alzheimer disease. Cell Rep. 37, 110158 (2021).
-
Norris, G. T. & Kipnis, J. Immune cells and CNS physiology: microglia and beyond. J. Exp. Med. 216, 60–70 (2019).
-
Chen, X. et al. Transcriptomic mapping uncovers Purkinje neuron plasticity driving learning. Nature 605, 722–727 (2022).
-
Da Mesquita, S., Fu, Z. & Kipnis, J. The meningeal lymphatic system: a new player in neurophysiology. Neuron 100, 375–388 (2018).
-
Da Mesquita, S. et al. Meningeal lymphatics affect microglia responses and anti-Aβ immunotherapy. Nature 593, 255–260 (2021).
-
Rustenhoven, J. & Kipnis, J. Bypassing the blood-brain barrier. Science 366, 1448–1449 (2019).
-
Rustenhoven, J. et al. Functional characterization of the dural sinuses as a neuroimmune interface. Cell 184, 1000–1016 (2021).
-
Korn, T. & Kallies, A. T cell responses in the central nervous system. Nat. Rev. Immunol. 17, 179–194 (2017).
-
Siller-Farfan, J. A. & Dushek, O. Molecular mechanisms of T cell sensitivity to antigen. Immunol. Rev. 285, 194–205 (2018).
-
Loetscher, M. et al. Chemokine receptor specific for IP10 and mig: structure, function, and expression in activated T-lymphocytes. J. Exp. Med. 184, 963–969 (1996).
-
Madore, C., Yin, Z., Leibowitz, J. & Butovsky, O. Microglia, lifestyle stress, and neurodegeneration. Immunity 52, 222–240 (2020).
-
Gratuze, M. et al. Impact of TREM2R47H variant on tau pathology-induced gliosis and neurodegeneration. J. Clin. Invest. 130, 4954–4968 (2020).
-
Huang, Y. et al. Microglia use TAM receptors to detect and engulf amyloid beta plaques. Nat. Immunol. 22, 586–594 (2021).
-
Neefjes, J., Jongsma, M. L., Paul, P. & Bakke, O. Towards a systems understanding of MHC class I and MHC class II antigen presentation. Nat. Rev. Immunol. 11, 823–836 (2011).
-
Keren-Shaul, H. et al. A unique microglia type associated with restricting development of Alzheimer’s disease. Cell 169, 1276–1290 (2017).
-
Mathys, H. et al. Temporal tracking of microglia activation in neurodegeneration at single-cell resolution. Cell Rep. 21, 366–380 (2017).
-
Filiano, A. J. et al. Unexpected role of interferon-gamma in regulating neuronal connectivity and social behaviour. Nature 535, 425–429 (2016).
-
Khalil, M. et al. Neurofilaments as biomarkers in neurological disorders. Nat. Rev. Neurol. 14, 577–589 (2018).
-
Pardoll, D. M. The blockade of immune checkpoints in cancer immunotherapy. Nat. Rev. Cancer 12, 252–264 (2012).
-
Sharpe, A. H. & Pauken, K. E. The diverse functions of the PD1 inhibitory pathway. Nat. Rev. Immunol. 18, 153–167 (2018).
-
Rosenzweig, N. et al. PD-1/PD-L1 checkpoint blockade harnesses monocyte-derived macrophages to combat cognitive impairment in a tauopathy mouse model. Nat. Commun. 10, 465 (2019).
-
Baruch, K. et al. PD-1 immune checkpoint blockade reduces pathology and improves memory in mouse models of Alzheimer’s disease. Nat. Med. 22, 135–137 (2016).
-
Kumagai, S. et al. The PD-1 expression balance between effector and regulatory T cells predicts the clinical efficacy of PD-1 blockade therapies. Nat. Immunol. 21, 1346–1358 (2020).
-
Turley, S. J., Fletcher, A. L. & Elpek, K. G. The stromal and haematopoietic antigen-presenting cells that reside in secondary lymphoid organs. Nat. Rev. Immunol. 10, 813–825 (2010).
-
Kigerl, K. A., de Rivero Vaccari, J. P., Dietrich, W. D., Popovich, P. G. & Keane, R. W. Pattern recognition receptors and central nervous system repair. Exp. Neurol. 258, 5–16 (2014).
-
Huynh, T. V. et al. Lack of hepatic apoE does not influence early Aβ deposition: observations from a new APOE knock-in model. Mol. Neurodegener. 14, 37 (2019).
-
Noguchi, T. et al. Temporally distinct PD-L1 expression by tumor and host cells contributes to immune escape. Cancer Immunol. Res. 5, 106–117 (2017).
-
Wozniak, D. F. et al. Motivational disturbances and effects of L-dopa administration in neurofibromatosis-1 model mice. PLoS ONE 8, e66024 (2013).
-
Yuede, C. M. et al. Behavioral consequences of NMDA antagonist-induced neuroapoptosis in the infant mouse brain. PLoS ONE 5, e11374 (2010).
-
Khuchua, Z. et al. Deletion of the N-terminus of murine map2 by gene targeting disrupts hippocampal ca1 neuron architecture and alters contextual memory. Neuroscience 119, 101–111 (2003).
-
Hammond, T. R. et al. Single-cell RNA sequencing of microglia throughout the mouse lifespan and in the injured brain reveals complex cell-state changes. Immunity 50, 253–271 (2019).
Acknowledgements
We thank X. Zhang and S. Li for advice on scRNA-seq analysis; J. Rustenhoven, B. Korin and A. Rolls for advice on meninges isolation; D. Bender for assistance with multiplex immune monitoring; D. Gate for advice on T cell immunohistochemistry on human samples; N. Saligrama for advice on TCR and antigen analysis; and M. Gratuze for PLX3397 drug formulation. We thank the Department of Pathology and Immunology Flow Cytometry and Fluorescence Activated Cell Sorting Core for help with cell sorting. This work was supported by a Carol and Gene Ludwig Award for Neurodegeneration Research (D.M.H.), National Institute of Health grant NS090934 (D.M.H.), the JPB Foundation (D.M.H.), Cure Alzheimer’s Fund (D.M.H.) and Rainwater Charitable Foundation (D.M.H.). M.F. was supported by the Ministry of Science and Higher Education of the Russian Federation (agreement no. 075-15-2022-301). Single-nucleus sequencing was carried out at the McDonnell Genome Institute. Confocal microscopic analyses were carried out at the Washington University Center for Cellular Imaging supported by Washington University School of Medicine, The Children’s Discovery Institute of Washington University and St Louis Children’s Hospital (CDI-CORE-2015-505 and CDI-CORE-2019-813) and the Foundation for Barnes-Jewish Hospital (3770 and 4642). We thank E. Reiman, G. Serrano and T. Beach for human brain tissue. The schematic representations of the fear conditioning behavioural paradigms in Extended Data Fig. 9i were created with BioRender.com.
Author information
Authors and Affiliations
Contributions
X.C. and D.M.H. conceived the study. X.C. designed the study, carried out the experiments, analysed the data and wrote the draft of the manuscript. M.M., M.B.F., J.H., J.K., X.B., I. Smirnov. and C.W. were blinded to sample IDs for brain volume and neurofilament light chain measurement. M.F., E.A., X.C., M.N.A. and J.D.U. analysed scRNA-seq data. X.C., H.H. and M.W.K. carried out microglia in vitro antigen presentation assay. X.C., and I. Shchukina carried out anti-PDCD1 acute treatment flow cytometry assay. C.M.Y. was blinded to sample IDs, and carried out and analysed the memory behavioural assay. D.M.H. supervised the overall research. X.C. and D.M.H. wrote the manuscript with comments from all authors.
Corresponding author
Ethics declarations
Competing interests
D.M.H. is an inventor on a patent licensed by Washington University to C2N Diagnostics on the therapeutic use of anti-tau antibodies. D.M.H. co-founded and is on the scientific advisory board of C2N Diagnostics. D.M.H. is on the scientific advisory board of Denali and Cajal Neuroscience and consults for Genentech and Alector. J.K. is a member of a scientific advisory group for PureTech. All other authors declare no competing interests.
Peer review
Peer review information
Nature thanks the anonymous reviewers for their contribution to the peer review of this work.
Additional information
Publisher’s note Springer Nature remains neutral with regard to jurisdictional claims in published maps and institutional affiliations.
Extended data figures and tables
Extended Data Fig. 1 ApoE4 exacerbates tau-mediated neurodegeneration.
(a) Representative image of 9.5-month A/PE4 mouse brain sections stained with an anti-amyloid-Aβ (Aβ) antibody and X-34. Scale bar = 500 μm. (b) Representative images of 6 and 9.5-month TE4 mouse brain sections stained with AT8 antibody. Scale bar = 500 μm. (c) Quantification of brain regional volumes of 9.5-month mice normalized to 6-month in b. TE4-6 months: n = 7, TE4-9.5 months: n = 13. ***p < 0.0001 for hippocampus (Hip) vs. cortex dorsal to the hippocampus (Ctx); amygdala (Amyg) vs. Ctx and entorhinal/piriform cortex (Ent) vs. Ctx. One-way ANOVA with Tukey’s post hoc test. (d) Quantification of the area covered by AT8 of 9.5-month TE4 mouse brain sections in b. TE4-6 months: n = 7, TE4-9.5months: n = 13. ***p < 0.0001 for Hip vs. Ctx; Amyg vs. Ctx and Ent vs. Ctx. One-way ANOVA with Tukey’s post hoc test. (e) Representative images of 6-month TE4, 9.5-month E4, and 9.5-month TE4 mouse brain sections stained with NeuN. Scale bar = 50 μm. (f) Thickness of granule cell layer of the DG in 9.5-month E3, TE3, E4, TE4 mice. (E3: n = 5, TE3: n = 6, E4: n = 15 and TE4: n = 13). *p = 0.0130 for TE3 vs. TE4. Two-way ANOVA with Tukey’s post hoc test. (g) Correlation between DG neuronal layer thickness and hippocampal volume. n = 39 biologically independent animals from f. Pearson correlation analysis. R2 = 0.8335, p < 0.0001. (h, i) Representative images of 9.5-month E4 and TE4 mouse brain sections stained with MBP. Scale bar = 500 μm in h. Scale bar = 100 μm in i. (j-l) Volumes of hippocampus, entorhinal/piriform cortex and posterior lateral ventricle in 9.5-month E3, TE3, E4, TE4 mice. (E3: n = 5, TE3: n = 6, E4: n = 15 and TE4 = 13). p = 0.0505 for TE3 vs. TE4 in comparing the volume of the hippocampus, *p = 0.0207 for TE3 vs. TE4 in comparing the volume of the posterior lateral ventricle. Two-way ANOVA with Tukey’s post hoc test. (m—o) Volumes of hippocampus, entorhinal/ piriform cortex and posterior lateral ventricle in 9.5-month TE3, TE4, A/PE4, 5xE4 male and female mice. (TE3-M: n = 6, TE3-F: n = 11, TE4-M: n = 13, TE4-F: n = 17, A/PE4-M: n = 7, A/PE4-F: n = 6, 5xE4-M: n = 6, 5xE4-F: n = 7). **p = 0.0087 for TE4 male vs. female entorhinal/piriform cortex volume. Two-way ANOVA with Tukey’s post hoc test. Data are mean ± s.e.m.
Extended Data Fig. 2 Immune cell composition in brain parenchyma and meninges.
(a) FACS sorting of CD45Total and /or CD45high cells from brain parenchyma and meninges from A/PE4 mice for single cell immune RNA-seq. (b) Analysis of the CD4 and CD8 positive T cells present in the brain of E4 and TE4 mice by flow cytometry. (E4: n = 8, TE4: n = 14) Data are mean ± s.e.m., ***p < 0.0001, Unpaired two-tailed Student’s t test. (c) Representative cell type specific makers in brain parenchyma (CD45Total) clusters. (d) CD45high immune cells from parenchyma assigned into 12 cell types as visualized by UMAP plot. (e) Representative cell type specific makers in meninges (CD45Total).
Extended Data Fig. 3 T cell infiltration in the brain parenchyma with significant tauopathy.
(a) Representative flow cytometry gating plot of splenic lymphocytes. (b) Quantification of the proportion of indicated lymphocytes and their subsets among 9.5-month E4, A/PE4, and TE4 mice. (E4: n = 4, A/PE4: n = 4, TE4: n = 8). p = 0.648, 0.492, 0.992 for E4 vs. A/PE4; E4 vs. TE4; A/PE4 vs. TE4 in T cells; p = 0.614, 0.518, 0.089 for E4 vs. A/PE4; E4 vs. TE4; A/PE4 vs. TE4 in CD4+ T cells; p = 0.665, 0.719, 0.196 for E4 vs. A/PE4; E4 vs. TE4; A/PE4 vs. TE4 in CD8+ T cells. Two-way ANOVA with Tukey’s post hoc test. p = 0.629, 0.472, 0.095 for E4 vs. A/PE4; E4 vs. TE4; A/PE4 vs. TE4 in Treg. One-way ANOVA with Tukey’s post hoc test. (c) Quantification of CD3+ T cell number per DG area with 0.3 mm2 in 9.5 month E3, TE3, E4, TE4 mice. (E3: n = 5, TE3: n = 6, E4: n = 15 and TE4: n = 13). Two-way ANOVA with Tukey’s post hoc test. p = 0.1342 for TE3 vs. TE4. (d) Representative images of 9.5-month old E4, TE4, A/PE4 and 5xE4 mouse brain sections stained with CD3, Iba1, Aβ and X-34. Scale bar = 20 μm. Images are representative of results from n = 4 in E4, A/PE4 and TE4 respectively. (e) TEM image demonstrating presence of a cell with T cell like features in brain parenchyma of 9.5 month of TE4 mouse. Scale bar = 2 μm. Images are representative of results from n = 3 in TE4 mice.
Extended Data Fig. 4 Characterization of T cell populations within the parenchyma and meninges of mice with amyloid or tau pathology.
(a) Heatmap showing identified marker genes in each of the categorized cell types in Total T cells, CD4+ and CD8+ T cells. (b) Total T cells from brain parenchyma and meninges with TCR assigned into 13 cell types as visualized by UMAP plot. (c) TRAV and TRBV enrichment in CD8+ and CD4+ T cells in TE4 and A/PE4 mice. (d) Representative TCR-TRBV projection in E4, A/PE4 and TE4 mice.
Extended Data Fig. 5 Expression of cytokines, chemokines, growth factors and soluble receptors in brain lysates.
Quantification of cytokines, chemokines, growth factors and soluble receptors in brain lysates in 9.5 month old E4, TE4, and TEKO mice. (E4: n = 10, TE4: n = 10 and TEKO: n = 10). One-way ANOVA with Tukey’s post hoc test. With Q=0.1% identify outlier function, n = 1 E4 and n = 1 TEKO samples for IFN-γ measurements were removed; n = 1 E4 sample for IL-1β measurements was removed. ***p = 0.0001, ***p < 0.0001, ***p < 0.0001, ***p < 0.0001, p = 0.1261, ***p < 0.0001, **p = 0.0038, ***p = 0.0005, ***p < 0.0001, *p = 0.0144, **p = 0.0022, ***p < 0.0001, *p = 0.0126, ***p = 0.0002, ***p = 0.0001, **p = 0.0074, **p = 0.0059, *p = 0.0434, ***p < 0.0001, **p = 0.007, *p = 0.038, ***p < 0.0001 for E4 vs. TE4 following the panel order. p = 0.2757, ***p < 0.0001, ***p < 0.0001, ***p < 0.0001, *p = 0.489, ***p < 0.0001, p = 0.2539, p = 0.9952, **p = 0.0011, p = 0.053, n = 0.625, ***p < 0.0001, n = 0.7867, p = 0.1013, p = 0.087, p = 0.9905, p = 0.1107, p = 0.7159, p = 0.9193, p = 0.8426, p = 0.83, **p = 0.0076 for TE4 vs. TEKO following the panel order. Data are mean ± s.e.m.; One-way ANOVA with Tukey’s post hoc test.
Extended Data Fig. 6 Changes in microglia and T cells with Tau-meditated neurodegeneration require ApoE.
(a) MHCII, CD206, Iba1 and GFAP staining in 9.5-month TE4 mice. Scale bar = 100 μm. Images are representative of results from n = 13 in TE4 mice. (b) Iba1, MHCII and Aβ staining in 9.5 month E4, A/PE4, TE4 and TEKO mice in Cortex dorsal to Hippocampus, Hippocampus and Ent/Piri cortex. Scale bar = 50 μm. (c) Quantification of the area covered by MHCII in Ctx, Hip and Ent in 9.5-month TE4 and TEKO mice. (TE4: n = 13 and TEKO: n = 15). ***p < 0.0001 for TE4-Hip vs. TEKO-Hip. One-way ANOVA with Tukey’s post hoc test. (d) Iba1, CD11c and Aβ staining in 9.5-month E4, A/PE4, TE4 and TEKO mice in Prefrontal cortex, Hippocampus and Ent/Piri cortex. Scale bar = 50 μm. (e) Quantification of the area covered by CD11c in Ctx, Hip and Ent in 9.5-month TE4 and TEKO mice. (TE4: n = 13 and TEKO: n = 15). ***p < 0.0001 for TE4-Hip vs. TEKO-Hip. One-way ANOVA with Tukey’s post hoc test. (f) Volume of hippocampus in 9.5-month TE4 and TEKO mice. (TE4: n = 13 and TEKO: n = 15). ***p < 0.0001. Unpaired two-tailed Student’s t test. (g) Quantification of numbers of CD3+ T cells in DG per 0.3 mm2. (TE4: n = 13 and TEKO: n = 15). ***p < 0.0001. Data are mean ± s.e.m.; Unpaired two-tailed Student’s t test.
Extended Data Fig. 7 IFN-γ in the T cell population and microglia can directly present antigen to CD8+ T cells in vitro.
(a) Ligand-receptor analysis in T cells and microglia. (b) IFN-γ expression in brain parenchyma (CD45Total) 12 cell types and T cells from brain parenchyma 15 clusters of T cells as visualized by UMAP plot. (c) The gating strategy for sorting naïve OT-1 CD8+ T cells, dendritic cells (DCs), and microglia. (d) Representative flow cytometry plot to assess the proliferation of OT-1 T cells by cell tracer violet (CTV) dilution after 3 days of co-culture with APCs in the presence of OVA. (e) Representative flow cytometry plot showing dose dependent OVA antigen presentation by DCs, microglia, or microglia in the presence of IFNγ assayed by OT-1 proliferation. (f) Percent of proliferating OT-1 T cells under the indicated conditions. Data are from one representative experiment. Two independent experiments were done showing similar results.
Extended Data Fig. 8 Blocking IFNγ signaling reduces tau-mediated neurodegeneration and tau pathology.
(a) Representative images of 9.5-month TE3-IgG and TE3-αIFN-γ treated mouse brain sections stained with Sudan black. Scale bar = 1 mm. (b–d) Volumes of hippocampus, entorhinal/piriform cortex and posterior lateral ventricle in 9.5-month TE3-IgG and TE3-αIFNγ treated mice. (TE3-IgG: n = 12 and TE3-αIFNγ: n = 12). p = 0.0479, 0.0398, 0.265 for TE3-IgG vs. TE3-αIFNγ in comparing the volumes of hippocampus, entorhinal/piriform cortex and posterior lateral ventricle, respectively. Unpaired two-tailed Student’s t test. (e) CD11c, CD8, Iba1 staining in 9.5-month TE3-IgG and TE3-αIFNγ treated mice. Scale bar = 50 μm. (f) Quantification of area covered by CD11c in 9.5-month TE3-IgG and TE3-αIFNγ mice. (TE3-IgG: n = 12 and TE3-αIFNγ: n = 12). *p = 0.0344. Unpaired two-tailed Student’s t test. (g) Representative images of 9.5-month TE3-IgG and TE3-αIFNγ treated mouse brain sections stained with AT8 antibody. Scale bar = 250 μm. (h) p-Tau (AT8) covered area in 9.5-month TE3-IgG and TE3-αIFNγ treated mice. (TE3-IgG: n = 12 and TE3-αIFNγ: n = 12). *p = 0.0122. Unpaired two-tailed Student’s t test. (i) Schematic representation of the timeline of PLX3397 treatment for microglia depletion. (j) Schematic representation of the timeline of anti-CD4 and anti-CD8 antibody treatment for T cell depletion.
Extended Data Fig. 9 T cell depletion in tauopathy mice.
(a) Representative flow cytometry gating plot and quantification of CD4+, CD8+ T cells in brain parenchyma, meninges and blood in IgG control or α-CD4 and α-CD8 (αT) treated mice. (b) Bar plot showing the number of CD8+ T and CD4+ T cells in IgG and αT treated mice. (IgG: n = 2 and αT: n = 4). Data are mean ± s.e.m. (c) Microglia from brain parenchyma of TE4-IgG and TE4-αT treated mice assigned into 3 categories as visualized by UMAP plot. (d) Bar plot showing the percentage of the 3 categories of microglia in TE4-IgG and TE4-αT mice. (e) Heat map showing representative functional genes specifically expressed in active microglia clusters in TE4-IgG and TE4-αT mice. (f) Quantification of nest-building behavior at 9.5 months age. (TE4-IgG: n = 15 and TE4-αT: n = 21). *p = 0.02 for IgG vs. αT. Fisher’s exact test. (g) Quantification of total rearing at baseline levels of general exploratory behavior in 1 h. (TE4-IgG: n = 10 and TE4-αT: n = 15). p = 0.4363 for TE4-IgG vs. TE4-αT. Unpaired two-tailed Student’s t test. (h) Quantification of total ambulations at baseline levels of locomotor activity levels in 1 h. (TE4-IgG: n = 10 and TE4-αT: n = 15). p = 0.0709. Two-tailed Mann-Whitney test. (i) Schematic representation of fear conditioning behavioral paradigms. Schematics in i were created with BioRender.com.
Extended Data Fig. 10 Blocking PD-1 immune checkpoint increases Foxp3+CD4+ Tregs and reduces tau-mediated neurodegeneration.
(a) The gating strategy for sorting Foxp3+ CD4+ Treg, Pd1+ Foxp3+ CD4+ Treg, Klrg1+ effector CD8+ T cells, PD-1+ Tox1+ CD8+ exhausted T cells in the brain parenchyma. (b–e) Quantification of T cell populations in the brain parenchyma of mice acutely treated with IgG control and αPD-1 antibodies. (TE4-IgG: n = 12 and TE4-αPD-1: n = 13 for b, d, e; TE4-IgG: n = 10 and TE4-αPD-1: n = 11 for c). *p = 0.041, **p = 0.0075, p = 0.52, p = 0.945 for Foxp3+CD4+ Treg, Pd1+ Foxp3+ CD4+ Treg, Klrg1+ effector CD8+ T cells, PD-1+ Tox1+ CD8+ exhausted T cells. Unpaired two-tailed Student’s t test. (f) Representative images of 9.5-month TE4-IgG and TE4-αPD-1 treated mouse brain sections stained with Sudan black. Scale bar = 1 mm. (g-h) Volumes of hippocampus, entorhinal/piriform cortex in 9.5-month TE4-IgG and TE4-αPD-1 treated mice. (TE4-IgG: n = 14 and TE4-αPD-1: n = 14). *p = 0.018 and 0.015 for TE4-IgG vs. TE4-αPD-1 in comparing the volumes of hippocampus, entorhinal/piriform cortex, respectively. Unpaired two-tailed Student’s t test. (i) Quantification of the area covered by AT8 in DG per slice in 9.5-month TE4-IgG vs. TE4-αPD-1 mice (TE4-IgG: n = 14 and TE4-αPD: n = 14). **p = 0.0009. Unpaired two-tailed Student’s t test.
Supplementary information
Supplementary Video 1
CD3 and IBA1 staining in hippocampus of TE4 mice. IBA1 (red) and CD3 (green) staining in 9.5-month-old TE4 mice with tau pathology in DG. Scale bar, 10 μm.
Supplementary Video 2
CD11c, CD8 and IBA1 staining in hippocampus of TE4 mice. IBA1 (blue), CD11c (red) and CD8 (green) staining in 9.5-month-old TE4 mice with tau pathology in DG. Scale bar, 15 μm.
Rights and permissions
Springer Nature or its licensor (e.g. a society or other partner) holds exclusive rights to this article under a publishing agreement with the author(s) or other rightsholder(s); author self-archiving of the accepted manuscript version of this article is solely governed by the terms of such publishing agreement and applicable law.
About this article
Cite this article
Chen, X., Firulyova, M., Manis, M. et al. Microglia-mediated T cell infiltration drives neurodegeneration in tauopathy. Nature (2023). https://ift.tt/iMRKw0J
-
Received:
-
Accepted:
-
Published:
-
DOI: https://ift.tt/iMRKw0J
Comments
By submitting a comment you agree to abide by our Terms and Community Guidelines. If you find something abusive or that does not comply with our terms or guidelines please flag it as inappropriate.
Health - Latest - Google News
March 08, 2023 at 11:17PM
https://ift.tt/L9KiFo5
Microglia-mediated T cell infiltration drives neurodegeneration in tauopathy - Nature.com
Health - Latest - Google News
https://ift.tt/36mxG5j
Bagikan Berita Ini
0 Response to "Microglia-mediated T cell infiltration drives neurodegeneration in tauopathy - Nature.com"
Post a Comment